Plants are continuously exposed to various environmental stresses that affect their growth, development, and yield. These stresses can be broadly categorized into abiotic and biotic factors. Abiotic stresses include drought, extreme temperatures, salinity, and nutrient deficiencies, while biotic stresses stem from pathogens, pests, and herbivory. To cope with these challenges, plants have evolved complex physiological, biochemical, molecular, and structural response mechanisms that allow them to survive and maintain productivity.
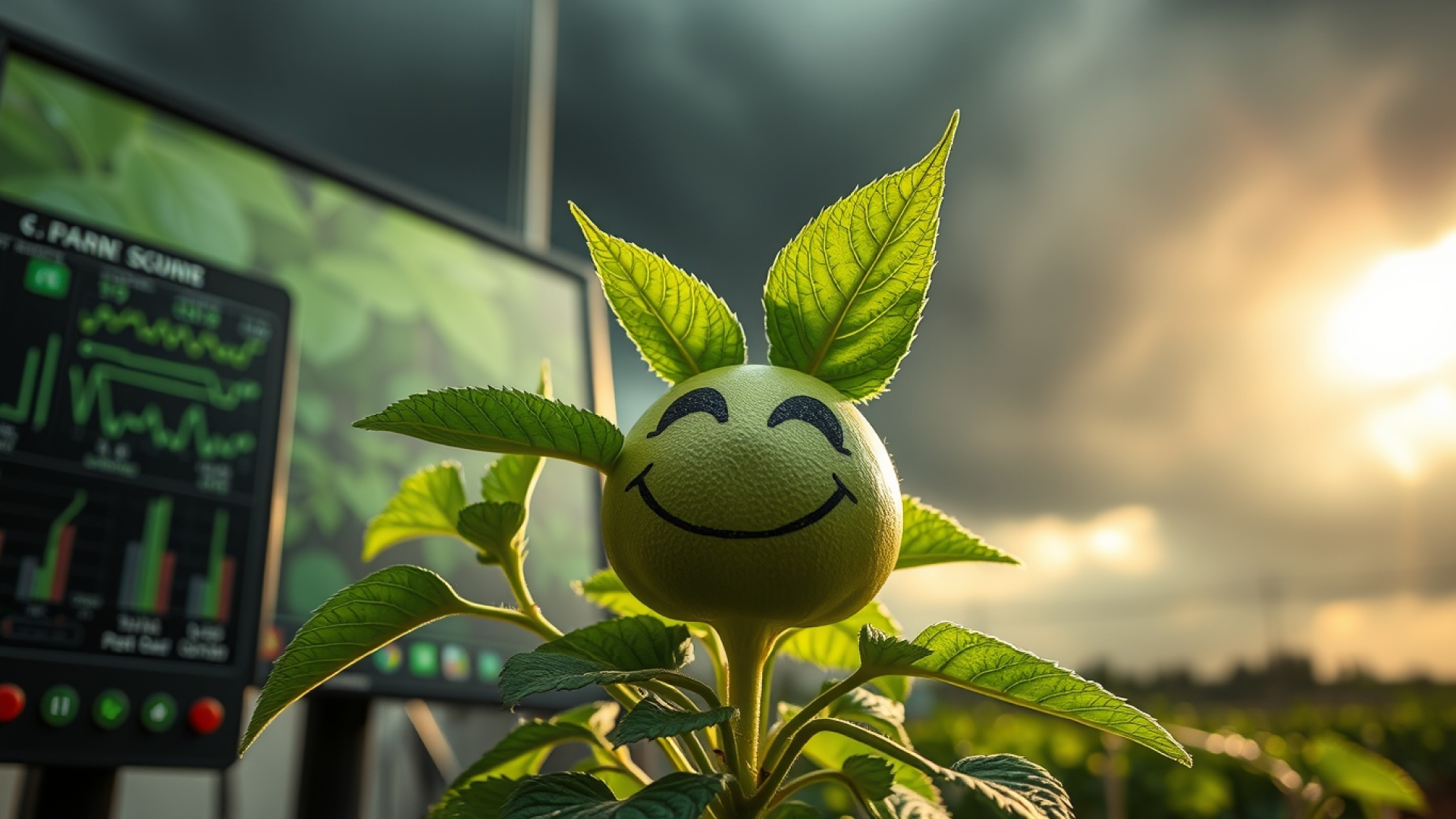
Plant Stress Responses
Plants employ multiple strategies to mitigate stress impacts. Physiological responses include changes in water regulation and metabolism. For instance, under drought conditions, plants close their stomata to reduce water loss through transpiration. They may also develop deeper root systems to access underground water reserves. During heat stress, plants synthesize heat shock proteins (HSPs) that protect cellular structures, whereas cold stress induces modifications in membrane fluidity by increasing the proportion of unsaturated fatty acids. In saline conditions, plants employ salt exclusion mechanisms or accumulate osmolytes such as proline and glycine betaine to maintain cellular homeostasis.
At the biochemical level, plants activate antioxidant defense systems to combat oxidative stress caused by an overproduction of reactive oxygen species (ROS). Enzymatic antioxidants, including superoxide dismutase (SOD), catalase (CAT), and ascorbate peroxidase (APX), play a crucial role in scavenging ROS and preventing cellular damage. Hormonal regulation also contributes to stress adaptation; for example, abscisic acid (ABA) mediates drought responses by controlling stomatal closure, while jasmonic acid (JA) and salicylic acid (SA) regulate defenses against insect herbivory and microbial pathogens, respectively. Ethylene, another key phytohormone, modulates stress responses by influencing senescence, fruit ripening, and defense mechanisms.
Molecular adaptations further enhance stress tolerance. Stress-responsive genes, such as those encoding dehydration-responsive element-binding (DREB) transcription factors, enhance drought resilience by regulating gene expression patterns. Epigenetic modifications, including DNA methylation and histone acetylation, enable plants to “remember” stress exposure, allowing for more efficient responses in subsequent generations.
Structural modifications also contribute to plant stress adaptation. The development of a thicker cuticle, the presence of trichomes, and changes in leaf morphology help minimize water loss and provide protection against herbivores. Additionally, plants may develop specialized structures such as salt glands to excrete excess salts in high-salinity environments.
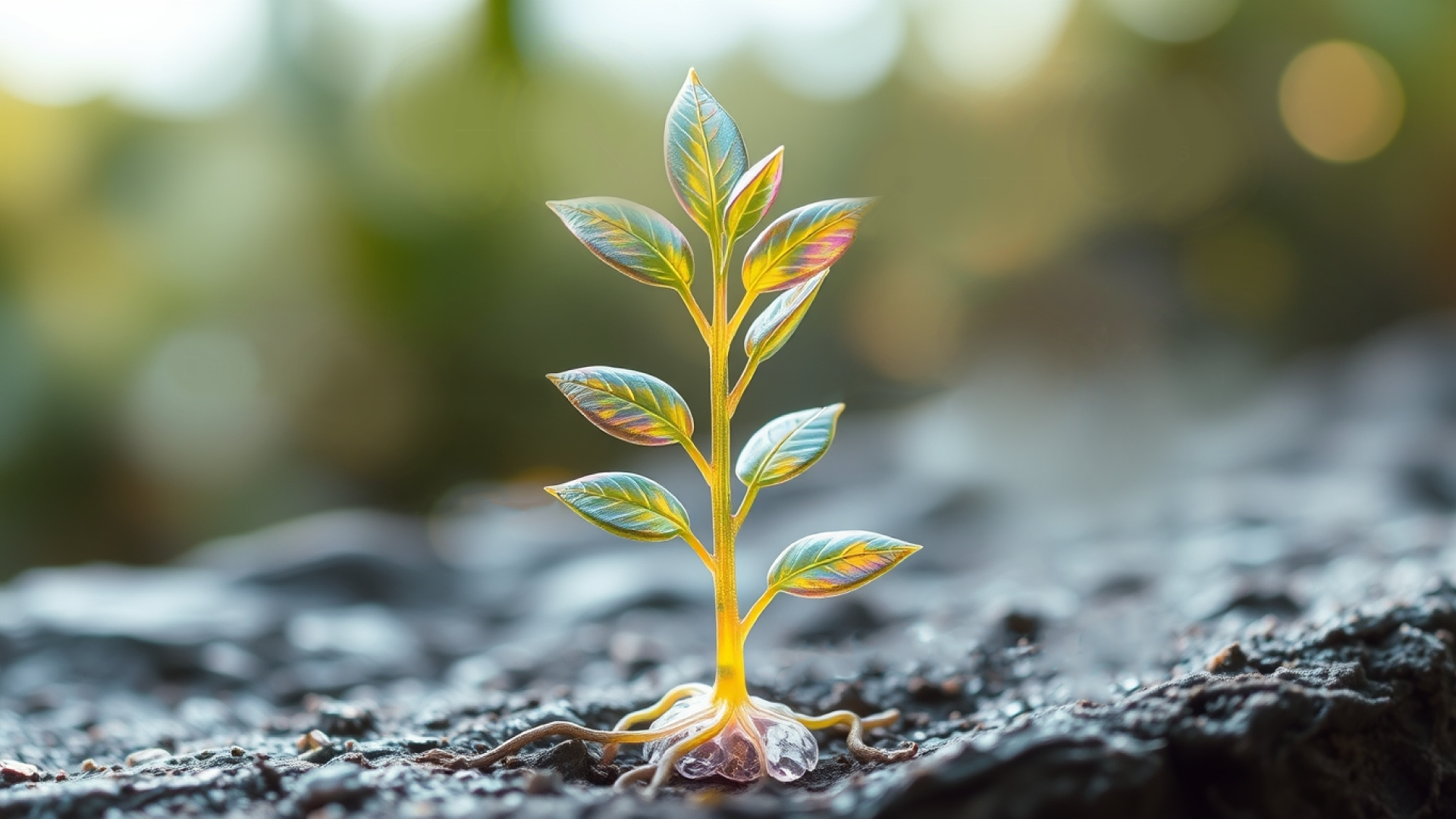
Detecting Plant Stress
Early detection of plant stress is crucial for effective intervention. Common symptoms include wilting, leaf curling, and chlorosis (yellowing) under drought conditions. Heat stress often leads to sunburn spots, leaf scorch, or premature flowering, while cold stress results in purpling, slowed growth, and frost damage. Salinity stress manifests through leaf tip burn, stunted growth, and salt deposits on the soil surface. Biotic stress, caused by pathogens and pests, results in symptoms such as necrotic lesions, holes, galls, and fungal mycelia on leaves and stems. However, visual identification often occurs too late, as irreversible damage may have already taken place.
To overcome these limitations, advanced sensor technologies enable the early detection of plant stress before symptoms become visible. These include multispectral and hyperspectral imaging, thermal cameras, fluorescence sensors, and AI-driven analysis.
AI and Sensor-Based Stress Detection
Multispectral and hyperspectral imaging, implemented via drones and satellites, provide insights into plant health by capturing reflectance data at different wavelengths. Healthy plants exhibit high reflectance in the near-infrared (NIR) region and strong absorption in the red and blue regions. Stress-induced changes alter these spectral characteristics, allowing for early detection. Vegetation indices, such as the Normalized Difference Vegetation Index (NDVI), track variations in plant vigor, while chlorophyll fluorescence (Fv/Fm) ratios indicate disruptions in photosynthetic efficiency. The Photochemical Reflectance Index (PRI) serves as an early indicator of stress by detecting carotenoid pigment changes associated with drought and heat stress.
Thermal imaging is another valuable tool for stress detection. Since drought-stressed plants reduce transpiration, their leaf temperatures increase. Infrared thermography can detect these temperature differences, enabling preemptive irrigation management before visible wilting occurs. Fluorescence imaging techniques, such as pulse-amplitude-modulated (PAM) fluorometry, assess chlorophyll fluorescence patterns to diagnose stress-induced disruptions in photosynthesis due to nutrient deficiencies, pathogen attacks, or environmental stressors.
Artificial intelligence (AI) enhances these sensor-based approaches by processing large datasets collected from remote sensing and in-field sensors. Machine learning algorithms, particularly convolutional neural networks (CNNs), analyze high-resolution images to identify subtle variations in leaf color, texture, and shape indicative of stress. AI-powered platforms, such as Google’s TensorFlow and IBM’s Watson AI, are increasingly being used for plant disease classification and stress prediction. Microsoft’s AI for Earth initiative is another example, utilizing AI models to analyze satellite imagery and monitor crop health on a global scale. Mobile applications, including Plantix and Agripredict, integrate AI-driven image recognition with real-time diagnostics, empowering farmers with instant stress assessment tools.
Future Trends in AI and Stress Detection
The integration of AI with the Internet of Things (IoT) is revolutionizing agriculture, leading to fully automated monitoring systems. Drones equipped with multispectral sensors and autonomous robots continuously collect and analyze plant health data, allowing for real-time stress detection and intervention. The development of edge AI, where AI models are embedded directly into field sensors, eliminates the need for cloud connectivity and enables instant on-site data analysis. The expansion of 5G networks is expected to further enhance real-time monitoring capabilities by enabling seamless communication between AI-driven devices and agricultural management platforms.
Another emerging trend is AI-assisted synthetic biology. By analyzing stress resilience data, AI can facilitate the development of genetically engineered crops with enhanced tolerance to drought, heat, and pest infestations. CRISPR-based gene-editing technologies, guided by AI-driven predictive models, have the potential to accelerate crop breeding programs and improve global food security.
Overall, AI and sensor-based plant stress detection technologies are transforming modern agriculture. By leveraging these advancements, farmers can optimize resource use, minimize yield losses, and ensure the resilience of food production systems in the face of climate change and environmental challenges. As AI continues to evolve, its applications in precision agriculture will play an increasingly vital role in sustainable farming practices and global food security.
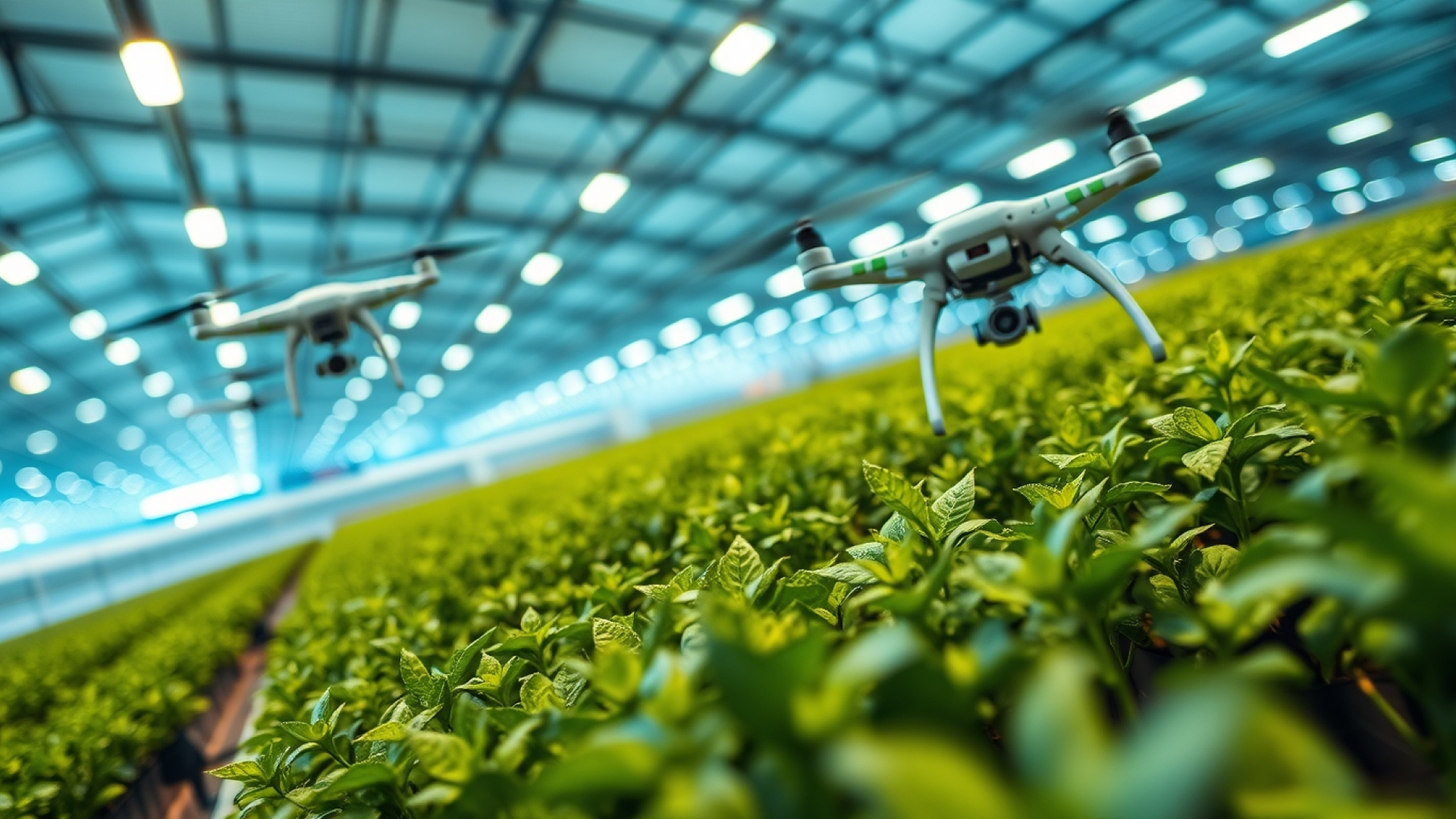